17 April 2024: Clinical Research
Muscular Function Recovery from General Anesthesia in 132 Patients Undergoing Surgery with Acceleromyography, Combined Acceleromyography, and Ultrasonography, and without Monitoring Muscular Function
Shiqian Huang123ACDEF, Yu Pan123BCD, Yu Wang123CDE, Shuaijie Pei123BCE, Xiang Wang4BC, Shujun Sun123AF, Shanglong Yao123DE, Xiangdong Chen123DE, Haifa Xia123ADG*DOI: 10.12659/MSM.942780
Med Sci Monit 2024; 30:e942780
Abstract
BACKGROUND: Diaphragmatic thickness fraction (DTF), measured by ultrasound, can predict the occurrence of postoperative residual neuromuscular blockade (RNMB). We hypothesized that the utilization of diaphragmatic ultrasound during the postoperative awakening phase of anesthesia in patients offers a successful means of avoiding RNMB in a notably comfortable manner, as compared to the use of acceleromyograph.
MATERIAL AND METHODS: Patients who underwent elective thyroid cancer radical surgery were enrolled in this prospective clinical study. Eligible participants were randomly assigned to 1 of 3 groups: 1) combined ultrasonography with acceleromyography group (the US+AMG group), 2) the AMG group, or 3) the usual clinical practice group (the UCP group). The primary outcomes of the study were the incidence of RNMB and hypoxemia after tracheal extubation.
RESULTS: The study included a total of 127 patients (43 in the US+AMG group, 44 in the AMG group, and 40 in the UCP group). The incidence of RNMB and hypoxemia was higher in the UCP group than in the US+AMG and AMG groups at 15 and 30 min after extubation, respectively. The mean area under the receiver operating characteristic curve, and the decision curve of the recovery rate of DTF (DTF) was greater than that of DTF.
CONCLUSIONS: The use of diaphragm ultrasound during the postoperative awakening phase of anesthesia can significantly reduce the incidence of RNMB. This method was non-inferior to the use of AMG during the entire perioperative period.
Keywords: Neuromuscular Blockade, Neuromuscular Monitoring, Ultrasonography
Introduction
Neuromuscular blocking drugs (NMBDs) are widely used in anesthesia practice, but their use has been associated with an important patient safety issue—postoperative residual neuromuscular blockade (RNMB) [1,2] – which has been reported to have an incidence as high as 60% [3]. Incomplete recovery of neuromuscular function can result in unpleasant muscle weakness symptoms, delayed extubation, postoperative pulmonary complications, re-intubation, and even anesthesia-related mortality [4,5].
Perioperative quantitative neuromuscular monitoring using acceleromyography (AMG) has been shown to be effective in preventing RNMB [6,7], but it is not routinely used in clinical practice [8]. This may be due to a lack of financial incentives, overconfidence of anesthesiologists in their knowledge and ability to manage NMBDs [9], and patient discomfort with train of four (TOF) stimuli [10]. Ultrasound measurements of the diaphragm in the zone of apposition have proven effective in visualizing the muscle and assessing its activity [11]. Diaphragm dysfunction can lead to severe adverse events and prolong mechanical ventilation [12,13], and be an indicator of respiratory effort in both critically ill and surgical patients [14,15]. Diaphragm dysfunction has been implicated in postoperative pulmonary complications with invasive ventilation [16]. Several recent studies have demonstrated that diaphragm thickening fraction (DTF) can serve as an index of successful extubation [14,15], weaning from mechanical ventilation [17], and the RNMB [18]. Nonetheless, DTF relies on individual characteristics such as height, weight, BMI, lung volume, and sedation. Our prior clinical observation revealed differences among patients in response to NMBDs [19], as well as baseline DTF. A prospective observational research study assessed the diagnostic accuracy of a series of ultrasound parameters in recognizing RNMB, using the TOF ratio (TOFr) as the reference standard [20]. Cappellini et al recently reported that the recovery rate of DTF (ΔTF) can better represent the condition of residual muscle relaxation in postoperative patients [18].
We hypothesized that combining diaphragmatic ultrasonography with AMG to monitor muscular function could effectively reduce the risk of RNMB compared to solely using AMG or without objective monitoring means. We conducted this clinical study to compare the incidence of RNMB and hypoxemia in patients receiving combined ultrasonography with AMG (the US+AMG group), the AMG group, and the usual clinical practice group (UCP group). We also evaluated the predictive performance of DTF and ΔTF for RNMB and hypoxemia.
Material and Methods
STUDY DESIGN AND PATIENTS:
This was a prospective clinical trial study, with a randomized parallel-group design, conducted at the Union Hospital of Tongji Medical College, Huazhong University of Science and Technology, and approved by Medical Ethics Committee of Tongji Medical College, Huazhong University of Science and Technology, Wuhan, China (S597). The study was conducted according to the guidelines laid down by the Declaration of Helsinki. Written informed consent for participation in this clinical study was obtained from all participants after taking sufficient time to think about it on the day before surgery. This study was registered in the Chinese Clinical Trial Registry on July 4, 2019, and the registration number was ChiCTR1900024270.
The study included patients who underwent elective thyroid cancer radical surgery and general anesthesia, with an anticipated surgery time of 1.5 to 4 h, aged between 18 and 65 years, with a body mass index (BMI) between 18.0 and 27.9 kg/m2, and an American Society of Anesthesiologists physical status (ASAPS) of I–II, and who signed the informed consent form. Exclusion criteria were severe cardiopulmonary, hepatic, or renal diseases; history of neurological disease; underlying neuromuscular disease or took medications that could affect neuromuscular function; conditions that could not allow for TOFr to be measured accurately by ulnar nerve stimulation; diaphragmatic palsy; or pregnant and breastfeeding women.
RANDOMIZATION AND BLINDING:
We randomly allocated 132 patients into 3 groups: the US+AMG group, the AMG group, and the UCP group. Randomization was based on the patient’s enrollment order and the random code generated in SAS statistical software (version 9. SAS Institute, Cary, NC, USA) using simple randomization. To minimize bias, data in the post-anesthesia care unit (PACU) were collected by an investigator who was blinded to the grouping, and the statistician was also blinded.
INTERVENTION PLAN:
A standardized anesthetic technique was administered without any premedication to reduce confounding factors among the 3 groups. 1) In the US+AMG group, AMG was utilized for continuous neuromuscular function monitoring throughout the surgery, with diaphragm ultrasound employed for monitoring before extubation in the PACU after the surgery. Extubation was performed when the ΔTF to 0.7 (based on the pre-experimental results) in US+AMG group. 2) The AMG group consistently used AMG for neuromuscular function monitoring throughout the entire perioperative period. Extubation was performed when the TOFr >0.9 in AMG group. 3) In the UCP group, extubation was performed by an experienced anesthesiologist based on clinical assessment, without specific neuromuscular function monitoring. The clinical assessments in the UCP group mainly included sustained head-lift, hand grip, and tongue depressor tests [21].
Baseline DTF was assessed using a Philips IU22 Color Doppler Ultrasound (Philips Ltd, Amsterdam, the Netherlands) by a specialist for each patient prior to anesthesia induction (refer to Figure 1). In the PACU, diaphragm ultrasound scans were carried out every 3 min for diaphragmatic thickness in US+AMG group, by a single, well-trained anesthesiologist. DTF can be calculated as the difference between the thickness at the end of inspiration (TEI) and the thickness at the end of expiration (TEE), as (TEI-TEE)/TEE. ΔTF was defined as the ratio of DTF to the corresponding baseline value.
AMG was employed to monitor the response of the adductor pollicis muscle (refer to Method S1). The AMG (TOF-Watch-SX; MSD BV Ltd, Oss, the Netherlands) was calibrated (CAL-2 mode) for baseline TOFr, after the administration of propofol (2.5 mg/kg ideal body weight) and sufentanil (0.4 μg/kg) but prior to initiating muscle relaxation with rocuronium (0.9 mg/kg). The response of the adductor pollicis muscle to a 50-mA TOF stimulation was recorded. The TOF stimulation was repeated every 30 s during the observation phase. The TOFr used for analysis was the normalized value divided by the baseline TOFr. TOFr in the recovery room was determined in the same way as that used in the operating room [22]. Neostigmine (0.05 mg/kg) and atropine (0.02 mg/kg) were administered when the TOF count showed more than 3 twitches or TOFr ≥0.4.
OUTCOMES:
The primary outcomes assessed were the incidence of RNMB and hypoxemia at 15, 30, 45, and 60 min after extubation. RNMB was defined as TOFr <0.9, and hypoxemia was defined as pulse oxygen saturation (SpO2) below 94%. Additionally, secondary outcomes included extubation time, TOFr, and SpO2 at the time of extubation. Extubation time was defined as the time interval from administration of the reversal agent to tracheal extubation. Safety indicators were assessed, including visual analog scale (VAS) pain score, Aldrete score, postoperative nausea and vomiting (PONV), respiratory complications [23], and cardiovascular adverse events in the PACU.
STATISTICAL ANALYSES AND SAMPLE SIZE CALCULATION:
Continuous data are described using mean and standard deviation (SD), and were analyzed by ANOVA or Kruskal-Wallis test, as appropriate. The categorical data are reported as number (percentage) and analyzed using the chi-square or Fisher exact test. Tukey post hoc analyses were performed for pairwise comparisons between the 3 groups. The Bonferroni corrections were implemented to control the type I error at 0.05, while evaluating the differences in the primary outcome 3 times. The Bonferroni-adjusted
Additional analyses were conducted on the data from the US+AMG group to explore potential correlations between changes in DTF, ΔTF, and TOFr using Spearman correlation analyses. Moreover, the predictive performance of DTF and ΔTF for the primary outcome was assessed by entering them into univariable logistic regression algorithms as predictors to estimate the risk of RNMB and hypoxemia occurrence. The area under the receiver operating characteristic curve (AUC) of these predictors (DTF and ΔTF) was calculated to evaluate their predictive accuracy. In addition, the clinical utility of the 2 predictors was evaluated using decision curve analysis (DCA). All computations were conducted in R environment version 4.2.0 (April, 2022).
According to the literature, the residual incidence of RNMB after neostigmine antagonism with and without muscle relaxation monitor is approximately 8% and 60%, respectively [6,24–26]. We assumed that the incidence of RNMB in the US+AMG group is not higher than that in the AMG group. To test this assumption, we set the significance level (α) to 0.05, the power (1-β) to 0.80, and the randomization ratio to 1: 1: 1. Considering the possibility of a 10% sample dropout rate, we determined that each group should contain 45 patients (135 in total). The sample size was calculated using PASS software 15.0.5.
Results
PARTICIPANTS:
From October 1, 2021 to June 30, 2022, a total of 135 consecutive patients were assessed for eligibility, with 3 patients being excluded. Ultimately, 132 patients participated in the study, and 127 patients completed the follow-up analysis, with 5 dropouts (Figure 2). The characteristics of all participants showed no statistically significant differences among the 3 groups in age, weight, ideal weight, height, BMI, sex, ASAPS, or baseline DTF (P>0.05; Table 1). The surgery duration, anesthesia duration, induced rocuronium dose, the total dose of propofol, sufentanil, remifentanil, rocuronium, neostigmine, and atropine did not significantly differ among the groups.
OUTCOMES:
Figure 3 shows the primary outcomes of the 3 groups. At 15 min after tracheal extubation, the UCP group had a higher incidence of RNMB (0.600, 95% CI: 0.448~0.752) compared to the US+AMG group (0.093, 95% CI: 0.006~0.180) and AMG group (0.114, 95% CI: 0.020~0.207). The UCP group also had a higher proportion of patients with a TOFr of 0.7 (7 out of 40, 18.0%) (Supplemantary Table 1). Additionally, the incidence of hypoxemia in the UCP group was higher than in the US+AMG group (0.575 vs 0) and the AMG group (0.575 vs 0.091). Supplementary Table 2 shows the data on hypoxemia, with various levels of severity. However, no statistically significant difference was found between the US+AMG group and the AMG group for RNMB and hypoxemia at 15, 30, and 45 min after extubation (Bonferroni-adjusted P>0.05).
The effective and safety indicators at the time of tracheal extubation are summarized in Supplementary Table 3. The extubation time was significantly shorter in the UCP group than in the US+AMG group (10 vs 8 min) and the AMG group (11 vs 8 min). However, no statistically significant difference was found between the US+AMG group and the AMG group for extubation time, TOFr, and SpO2 at extubation (Bonferroni-adjusted P>0.05). The differences in safety indexes, including Aldrete score, Ramsay score, VAS score for pain, and PONV incidence (%), were not statistically significant among the 3 groups (P>0.05). No patients experienced cardiovascular or respiratory complications (excluding hypoxemia) (Supplementary Table 3).
The results of the Spearman correlation coefficient analysis indicated a strong correlation between the levels of DTF, ΔTF, and TOFr (Figure 4A, 4B). In terms of predicting the primary outcomes (RNMB and hypoxemia), we constructed univariable logistic regression algorithms with DTF or ΔTF as the predictor variable. ΔTF showed superior predictive performance, with a cutoff value of 80.95%, a sensitivity of 1.00, and a specificity of 0.65, compared to DTF with a cutoff value of 43.52%, a sensitivity of 0.75, and a specificity of 0.53. The receiver operating characteristic curves corresponding to the 2 predictors (DTF and ΔTF) revealed that ΔTF had a higher AUC than DTF (0.814 vs 0.462), suggesting superior discrimination performance (Figure 4C). Based on the DCA of the 2 logistic models, the net benefit for ΔTF was significantly greater than that of DTF (Figure 4D).
Discussion
This prospective parallel-group clinical study demonstrated a significant decrease in the incidences of RNMB and hypoxemia in the US+AMG and AMG groups compared to the UCP group in the PACU. Additionally, we confirmed that ΔTF has a notably superior predictive capability for RNMB and hypoxemia compared to DTF. To the best of our knowledge, this is the first clinical study to use ΔTF detected by diaphragm ultrasound to guide postoperative extubation.
Despite the routine use of neostigmine to reverse RNMB after surgery, the incidence of RNMB in the UCP group was still alarmingly high, warranting further attention. Our investigation revealed that 15 min after tracheal extubation, the incidence of RNMB was 60.0% in the UCP group compared to 11.4% in the AMG group and 9.3% in the US+AMG group. These results align with the literature, where the incidence of RNMB fluctuated between 7.8% and 60%, although neostigmine was used as an antagonist to reverse muscle relaxation [24,26,27]. There is no clinical assessment, based on either experience or clinical indicators, with a satisfactory level of effectiveness to identify RNMB [28,29]. Sustained head-lift, hand grip, and tongue depressor tests have limited sensitivity (10–30%) and a best positive predictive value below 50% [30]. Therefore, monitoring muscle relaxation during the postoperative extubation period is essential for reducing the risk of RNMB. The strategy of using a combination of diaphragmatic ultrasound and AMG also demonstrated an effective monitoring value in a recent study focusing on critical respiratory events after extubation [31]. Murphy et al found that the incidence of hypoxemia on arrival in the PACU was lower in the AMG group than in the conventional or peripheral nerve stimulator group (SpO2 90–93%, 5.6% vs 24.4%; SpO2 <90%, 6.7% vs 43.3%), which is consistent with our findings [25].
DTF values greater than 30–36% have been shown to predict the success of extubation in spontaneous breathing trials [32,33]. A diagnostic test focusing on the use of ultrasonography parameters to recognize RNMB [20] suggested that the diaphragm excursion difference (DED) may have high specificity for the prediction of RNMB, and ultrasonography parameters are significantly correlated with the TOFr. We also observed a strong correlation between DTF, ΔTF, and TOFr in Spearman analyses. Furthermore, our regression analysis of the US+AMG subgroup indicated that ΔTF has superior predictive ability for RNMB and hypoxemia compared to DTF. Cappellini et al also found that ΔTF enabled observation of a significant difference in the recovery of baseline diaphragmatic function, which is consistent with our findings [18]. One potential explanation for this is that dividing the thickening fraction by the corresponding baseline value enables us to eliminate patient-related confounding factors. In fact, numerous studies have reported a wide range of inspiratory thickening of the diaphragm in healthy subjects, which depends on individual height, weight, BMI, and other characteristics [34,35].
The diaphragm, the primary muscle involved in breathing, has been implicated in postoperative pulmonary complications with invasive ventilation [16]. The development of diaphragmatic ultrasound allows for non-invasive direct observation of the movement of this essential muscle. The diaphragm, being the most resistant to NMBDs and the first to recover [36], may not be sufficiently sensitive for neuromuscular function monitoring. This limitation may explain why diaphragm monitoring has not gained widespread use in monitoring neuromuscular function. Nevertheless, diaphragmatic ultrasound offers several advantages in neuromuscular function monitoring compared to AMG, including comfort, convenience, and accuracy. Consequently, the preventive effect on RNMB achieved with diaphragmatic ultrasound may be non-inferior to that of AMG. Firstly, ultrasound-measured thickening fraction for diaphragmatic recovery after surgery is more comfortable for awake or soon-to-be-awakened patients than AMG. TOF stimuli can cause pain and agitation in awake patients, reducing the accuracy of AMG-derived TOF values [8]. Secondly, although neuromuscular monitoring programs with AMG may seem sensitive, users often have biases in interpreting the results due to various factors [37]. This has been exemplified by research showing that the validity of TOF at the adductor pollicis is frequently unstable [38–40]. A recent study reported that respiratory muscle strength could still be impaired even when the TOFr approaches 1.0, and ultrasound could estimate expiratory muscle strength from measurement of inspiratory muscle strength from diaphragmatic excursions [41]. Hence, AMG may not be the best method for neuromuscular monitoring, particularly in terms of accuracy and stability. The measurement of diaphragm thickening could be effective in detecting and preventing RNMB, warranting further investigation. Our results indicate that diaphragmatic ultrasound holds promise as an alternative to AMG for assessing muscular function before tracheal extubation. Our study found no significant difference in VAS scores among the 3 groups of patients, which may be due to the choice of surgical patients. Children, women, and others who are more sensitive to pain will benefit more from the non-invasive monitoring method of ultrasound. In view of this, we will further promote the application scenarios of diaphragm ultrasound in the future, from pre-extubation evaluation to post-extubation evaluation, and include surgical types such as gynecological surgery and pediatric surgery in the research scope.
However, our study is not without limitations. Firstly, the generalizability of our findings is limited due to the inherent limitations of randomized controlled trials. Further research is necessary to determine the diagnostic value of diaphragm ultrasound in populations at high risk for postoperative respiratory complications, such as elderly or obese patients. Moreover, the cutoff value for ΔTF as an indicator for tracheal extubation may vary among different sub-populations and requires further investigation. Another limitation of our study is the short observation period, as we focused only on early postoperative muscular function in the PACU. Future research should investigate longer-term outcomes, such as hospital stay and the occurrence of long-term complications.
Conclusions
The combination of AMG and diaphragm ultrasonography can be non-inferior to the method of AMG for perioperative monitoring of neuromuscular function. Additionally, our findings indicate that ΔTF value can serve as a suitable indicator for tracheal extubation to mitigate the risks associated with RNMB and hypoxemia.
Figures
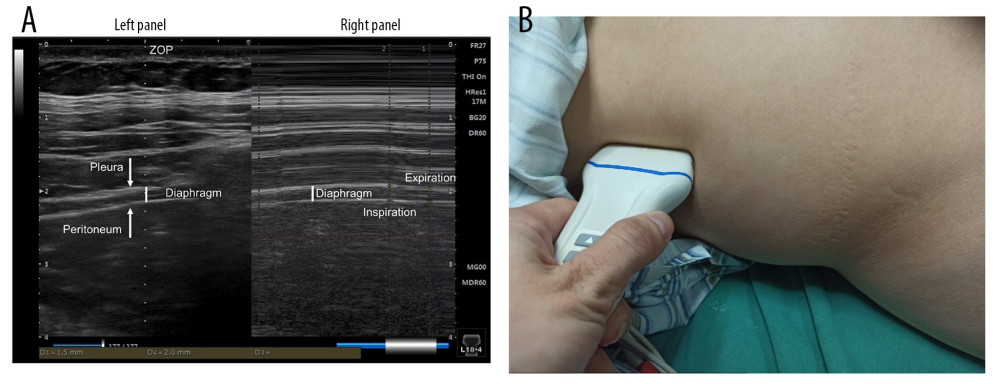
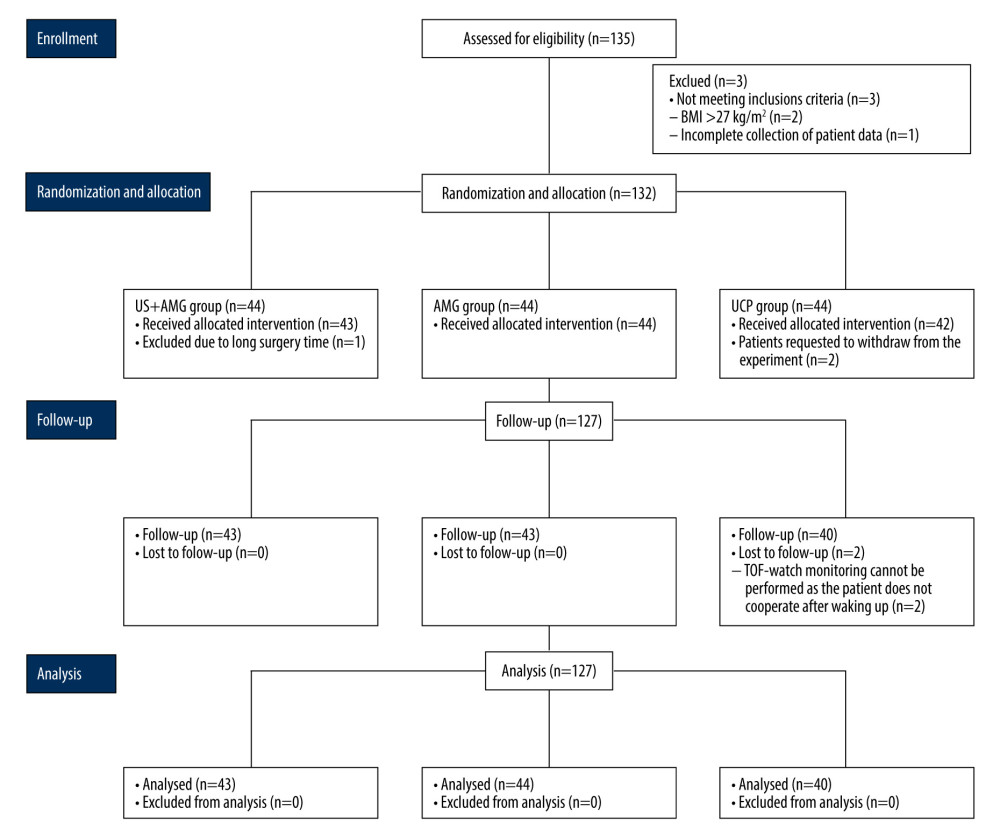
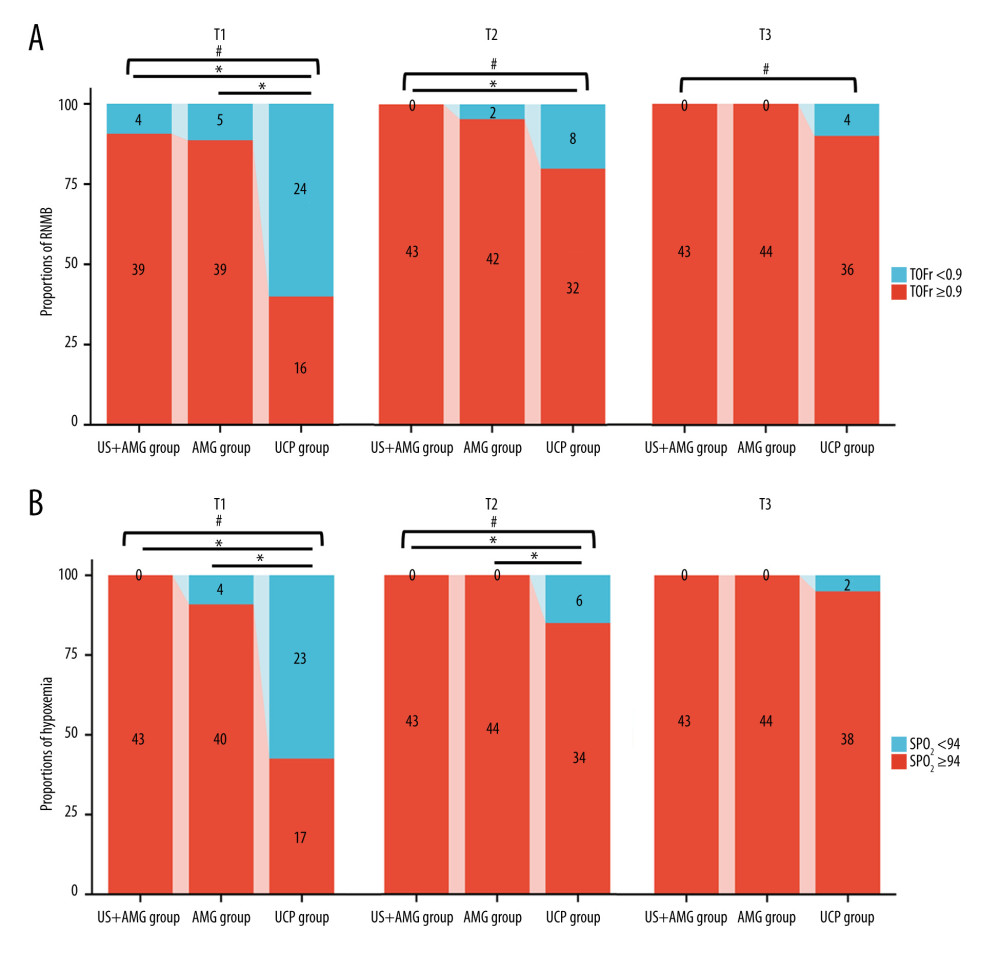
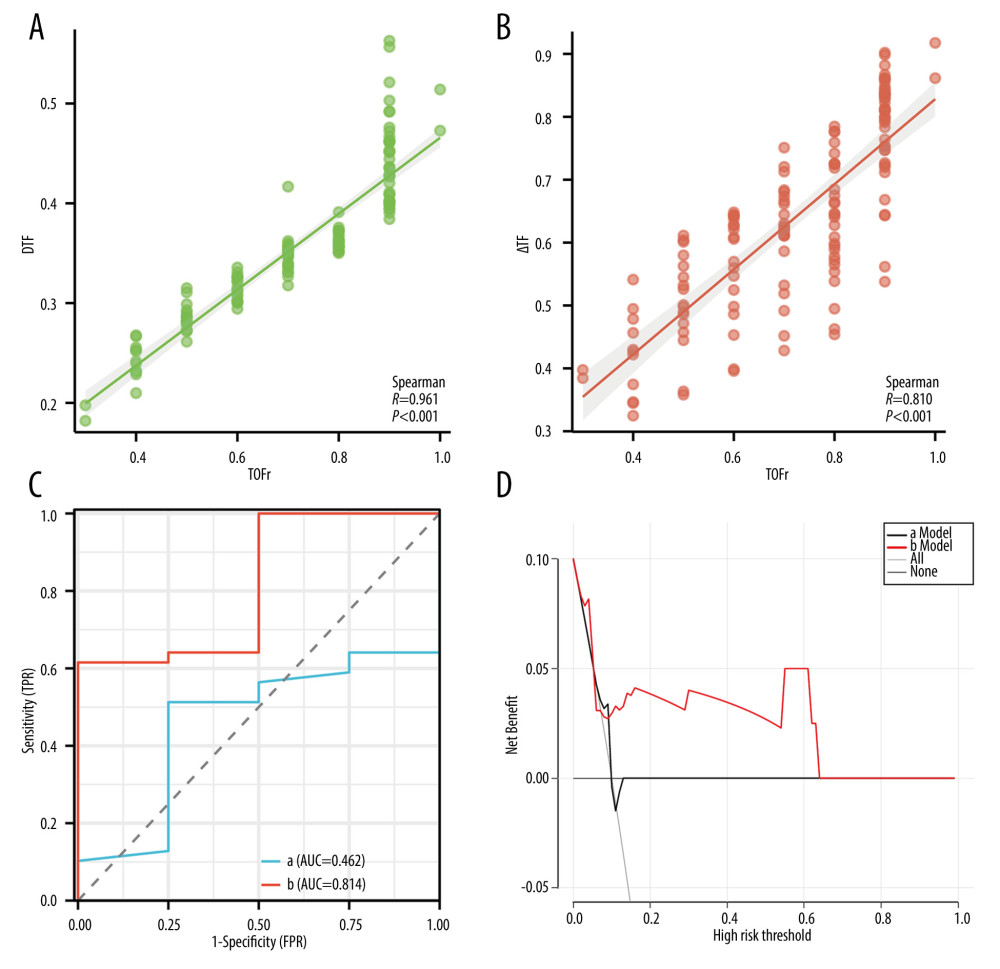
Tables
Table 1. Baseline, surgery, and anesthesia characteristics of participants in US+AMG group, AMG group, and UCP group.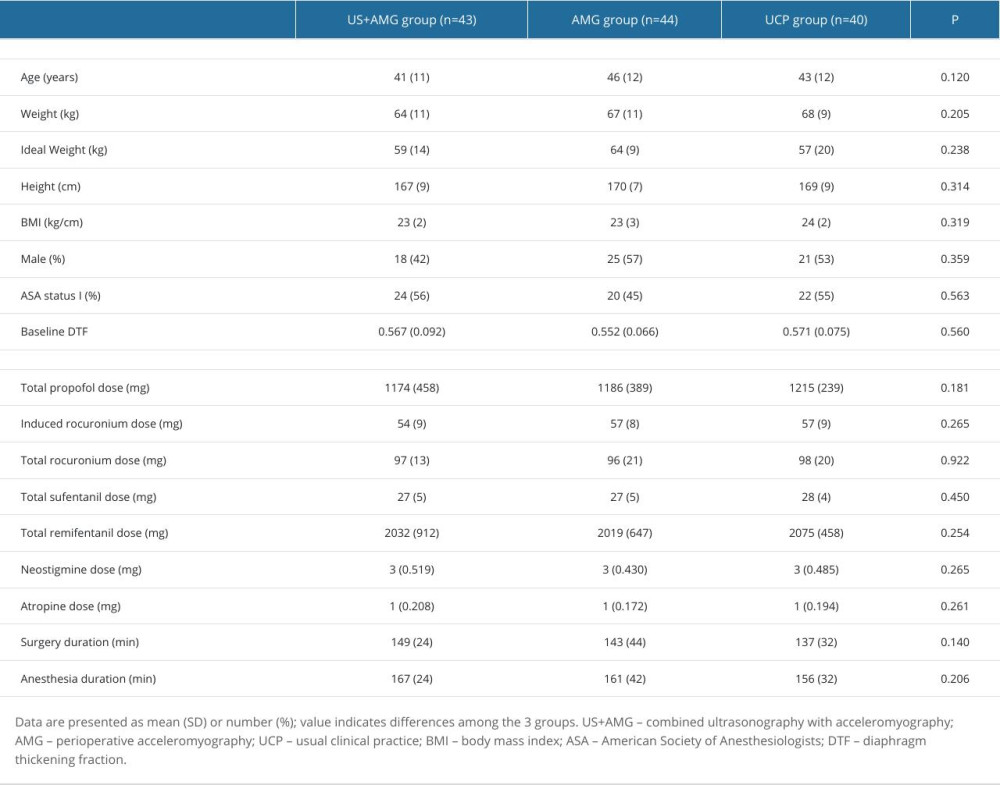
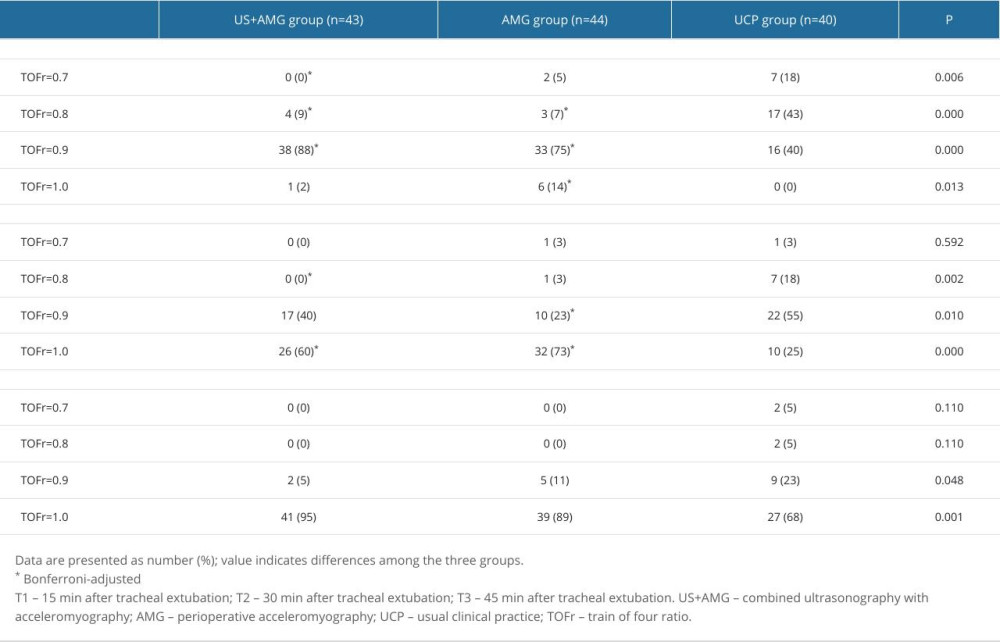
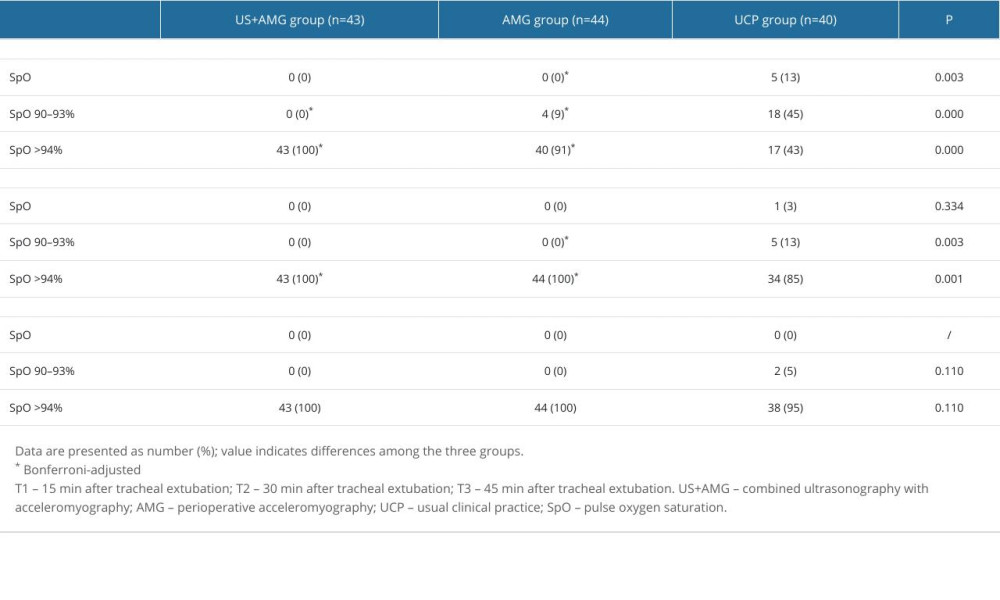
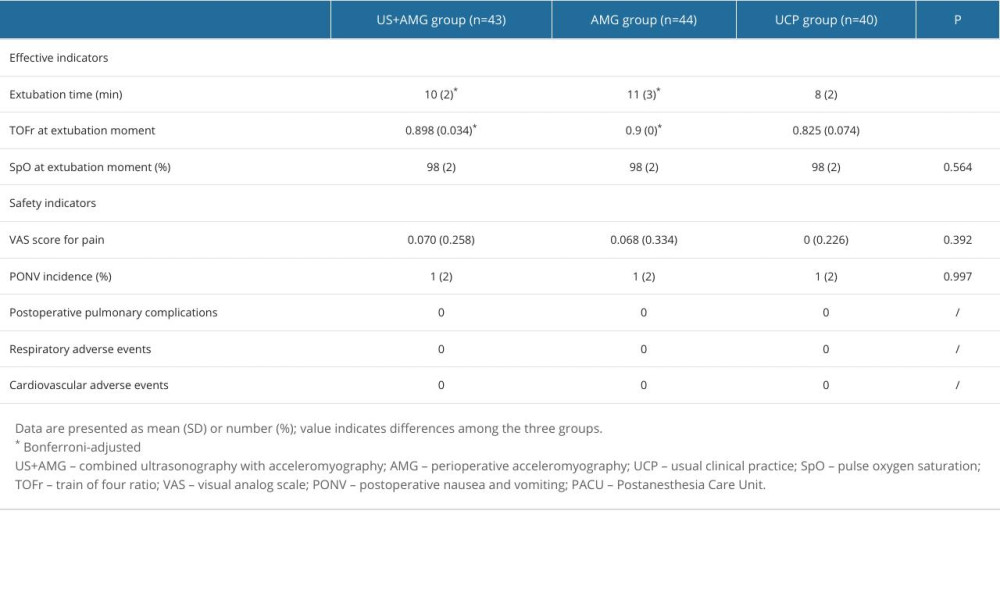
References
1. Yu B, Ouyang B, Ge S, Incidence of postoperative residual neuromuscular blockade after general anesthesia: A prospective, multicenter, anesthetist-blind, observational study: Curr Med Res Opin, 2016; 32(1); 1-9
2. Saager L, Maiese EM, Bash LD, Incidence, risk factors, and consequences of residual neuromuscular block in the United States: The prospective, observational, multicenter RECITE-US study: J Clin Anesth, 2019; 55; 33-41
3. Carvalho H, Verdonck M, Cools W, Forty years of neuromuscular monitoring and postoperative residual curarisation: A meta-analysis and evaluation of confidence in network meta-analysis: Br J Anaesth, 2020; 125(4); 466-82
4. Cedborg AI, Sundman E, Boden K, Pharyngeal function and breathing pattern during partial neuromuscular block in the elderly: Effects on airway protection: Anesthesiology, 2014; 120(2); 312-25
5. Nemes R, Fulesdi B, Pongracz A, Impact of reversal strategies on the incidence of postoperative residual paralysis after rocuronium relaxation without neuromuscular monitoring: A partially randomised placebo controlled trial: Eur J Anaesthesiol, 2017; 34(9); 609-16
6. Murphy GS, Szokol JW, Avram MJ, Intraoperative acceleromyography monitoring reduces symptoms of muscle weakness and improves quality of recovery in the early postoperative period: Anesthesiology, 2011; 115(5); 946-54
7. Murphy GS, Brull SJ, Quantitative neuromuscular monitoring and postoperative outcomes: A narrative review: Anesthesiology, 2022; 136(2); 345-61
8. Thomsen JL, Nielsen CV, Palmqvist DF, Premature awakening and underuse of neuromuscular monitoring in a registry of patients with butyrylcholinesterase deficiency: Br J Anaesth, 2015; 115(Suppl 1); i89-i94
9. Naguib M, Brull SJ, Hunter JM, Anesthesiologists’ overconfidence in their perceived knowledge of neuromuscular monitoring and its relevance to all aspects of medical practice: An international survey: Anesth Analg, 2019; 128(6); 1118-26
10. Baillard C, Bourdiau S, Le Toumelin P, Assessing residual neuromuscular blockade using acceleromyography can be deceptive in postoperative awake patients: Anesth Analg, 2004; 98(3); 854-57
11. Wait JL, Nahormek PA, Yost WT, Diaphragmatic thickness-lung volume relationship in vivo: J Appl Physiol (1985), 1989; 67(4); 1560-68
12. Luo L, Li Y, Chen X, Different effects of cardiac and diaphragm function assessed by ultrasound on extubation outcomes in difficult-to-wean patients: A cohort study: Bmc Pulm Med, 2017; 17(1); 161
13. Spadaro S, Grasso S, Dres M, Point of care ultrasound to identify diaphragmatic dysfunction after thoracic surgery: Anesthesiology, 2019; 131(2); 266-78
14. Umbrello M, Formenti P, Longhi D, Diaphragm ultrasound as indicator of respiratory effort in critically ill patients undergoing assisted mechanical ventilation: A pilot clinical study: Crit Care, 2015; 19(1); 161
15. Whitehead NP, Bible KL, Kim MJ, Validation of ultrasonography for non-invasive assessment of diaphragm function in muscular dystrophy: J Physiol, 2016; 594(24); 7215-27
16. Levine S, Nguyen T, Taylor N, Rapid disuse atrophy of diaphragm fibers in mechanically ventilated humans: N Engl J Med, 2008; 358(13); 1327-35
17. Parada-Gereda HM, Tibaduiza AL, Rico-Mendoza A, Effectiveness of diaphragmatic ultrasound as a predictor of successful weaning from mechanical ventilation: A systematic review and meta-analysis: Crit Care, 2023; 27(1); 174
18. Cappellini I, Ostento D, Loriga B, Comparison of neostigmine vs. sugammadex for recovery of muscle function after neuromuscular block by means of diaphragm ultrasonography in microlaryngeal surgery: A randomised controlled trial: Eur J Anaesthesiol, 2020; 37(1); 44-51
19. Zhu H, Wang Y, Wang Q, Polymorphisms contribute to differences in the effect of rocuronium in Chinese patients: Basic Clin Pharmacol Toxicol, 2022; 130(1); 141-50
20. Lang J, Liu Y, Zhang Y, Peri-operative diaphragm ultrasound as a new method of recognizing post-operative residual curarization: BMC Anesthesiol, 2021; 21(1); 287
21. Thilen SR, Weigel WA, Todd MM, 2023 American Society of Anesthesiologists Practice Guidelines for Monitoring and Antagonism of Neuromuscular Blockade: A Report by the American Society of Anesthesiologists Task Force on Neuromuscular Blockade: Anesthesiology, 2023; 138(1); 13-41
22. Feltracco P, Tonetti T, Barbieri S, Cisatracurium- and rocuronium-associated residual neuromuscular dysfunction under intraoperative neuromuscular monitoring and postoperative neostigmine reversal: A single-blind randomized trial: J Clin Anesth, 2016; 35; 198-204
23. Kirmeier E, Eriksson LI, Lewald H, Post-anaesthesia pulmonary complications after use of muscle relaxants (POPULAR): A multicentre, prospective observational study: Lancet Respir Med, 2019; 7(2); 129-40
24. Kumar GV, Nair AP, Murthy HS, Residual neuromuscular blockade affects postoperative pulmonary function: Anesthesiology, 2012; 117(6); 1234-44
25. Murphy GS, Szokol JW, Marymont JH, Intraoperative acceleromyographic monitoring reduces the risk of residual neuromuscular blockade and adverse respiratory events in the Postanesthesia Care Unit: Anesthesiology, 2008; 109(3); 389-98
26. Kotake Y, Ochiai R, Suzuki T, Reversal with sugammadex in the absence of monitoring did not preclude residual neuromuscular block: Anesth Analg, 2013; 117(2); 345-51
27. Kocaturk O, Kaan N, Kayacan N, The incidence of postoperative residual curarization following the use of intermediate-acting muscle relaxants and related factors: Middle East J Anaesthesiol, 2014; 22(6); 583-90
28. Brull SJ, Kopman AF, Current status of neuromuscular reversal and monitoring: Challenges and opportunities: Anesthesiology, 2017; 126(1); 173-90
29. Fuchs-Buder T, Romero CS, Lewald H, Peri-operative management of neuromuscular blockade: A guideline from the European Society of Anaesthesiology and Intensive Care: Eur J Anaesthesiol, 2023; 40(2); 82-94
30. Klein AA, Meek T, Allcock E, Recommendations for standards of monitoring during anaesthesia and recovery 2021: Guideline from the Association of Anaesthetists: Anaesthesia, 2021; 76(9); 1212-23
31. Huang L, Xia B, Cheng L, Use of a combination of diaphragmatic ultrasound and muscle relaxation monitoring in predicting post-extubation adverse respiratory events among elderly patients in an anesthesia intensive care unit: BMC Pulm Med, 2023; 23(1); 503
32. DiNino E, Gartman EJ, Sethi JM, Diaphragm ultrasound as a predictor of successful extubation from mechanical ventilation: Thorax, 2014; 69(5); 423-27
33. Ferrari G, De Filippi G, Elia F, Diaphragm ultrasound as a new index of discontinuation from mechanical ventilation: Crit Ultrasound J, 2014; 6(1); 8
34. Boon AJ, Harper CJ, Ghahfarokhi LS, Two-dimensional ultrasound imaging of the diaphragm: Quantitative values in normal subjects: Muscle Nerve, 2013; 47(6); 884-89
35. Goligher EC, Laghi F, Detsky ME, Measuring diaphragm thickness with ultrasound in mechanically ventilated patients: Feasibility, reproducibility and validity: Intensive Care Med, 2015; 41(4); 642-49
36. Nguyen-Huu T, Molgo J, Servent D, Resistance to D-tubocurarine of the rat diaphragm as compared to a limb muscle: Influence of quantal transmitter release and nicotinic acetylcholine receptors: Anesthesiology, 2009; 110(5); 1011-15
37. Harman A, Tung A, Fox C, Heuristics, overconfidence, and experience: Impact on monitoring depth of neuromuscular blockade: Anesth Analg, 2019; 128(6); 1057-59
38. Kopman AF, Klewicka MM, Neuman GG, The relationship between acceleromyographic train-of-four fade and single twitch depression: Anesthesiology, 2002; 96(3); 583-87
39. Todd MM, Hindman BJ, King BJ, The implementation of quantitative electromyographic neuromuscular monitoring in an academic anesthesia department: Anesth Analg, 2014; 119(2); 323-31
40. Capron F, Alla F, Hottier C, Can acceleromyography detect low levels of residual paralysis? A probability approach to detect a mechanomyographic train-of-four ratio of 0.9: Anesthesiology, 2004; 100(5); 1119-24
41. Huang C, Wang X, Gao S, Sugammadex versus neostigmine for recovery of respiratory muscle strength measured by ultrasonography in the postextubation period: A randomized controlled trial: Anesth Analg, 2023; 136(3); 559-68
Figures
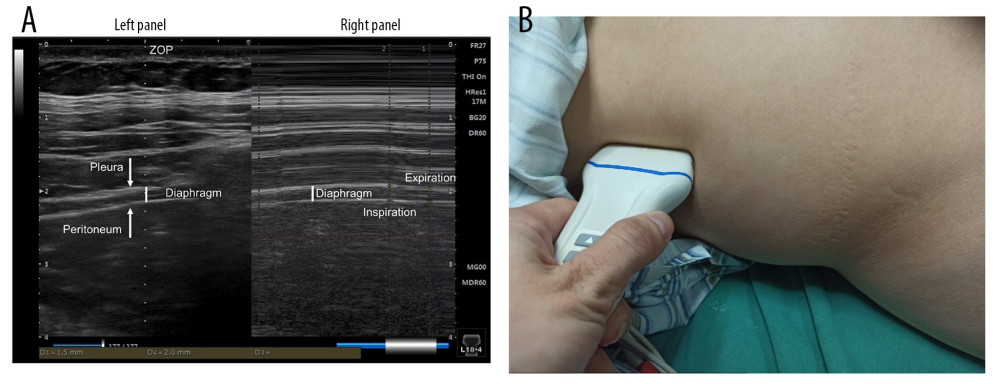
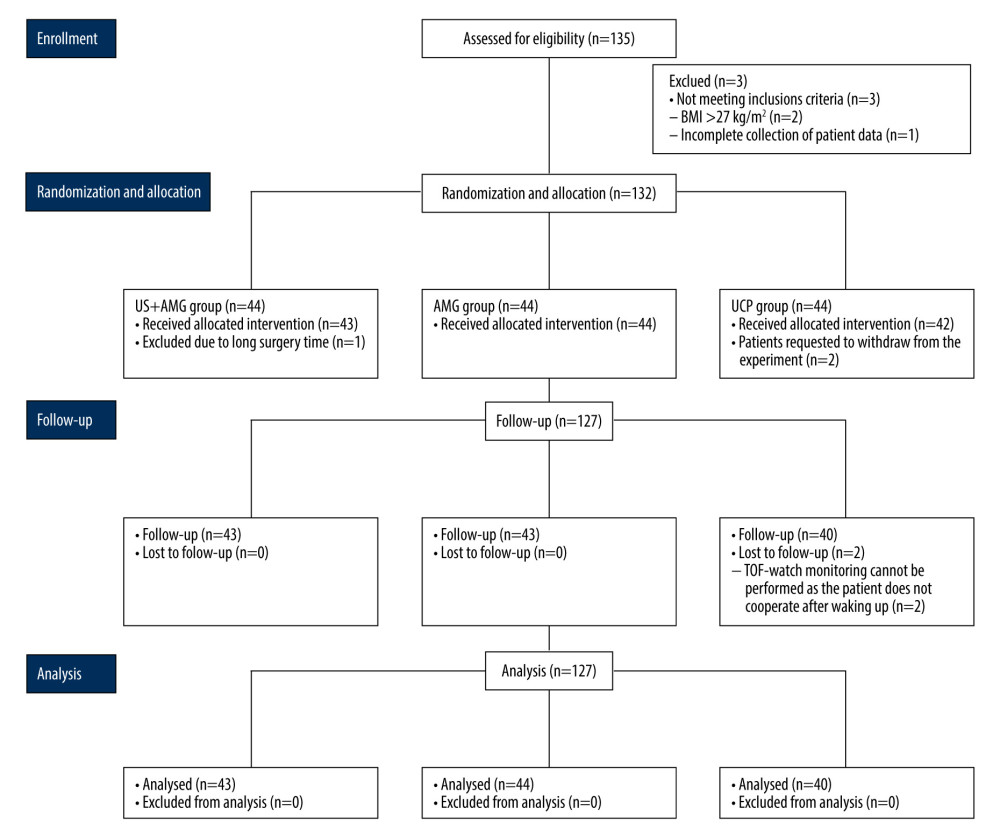
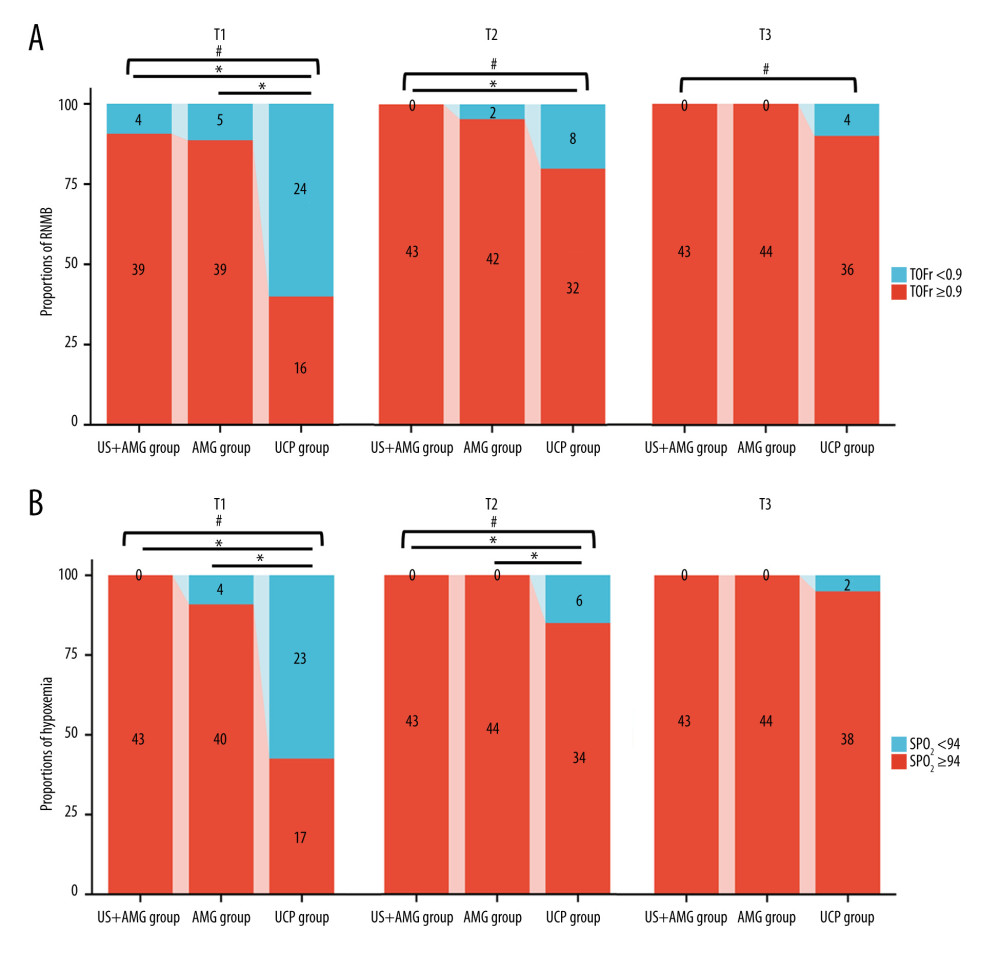
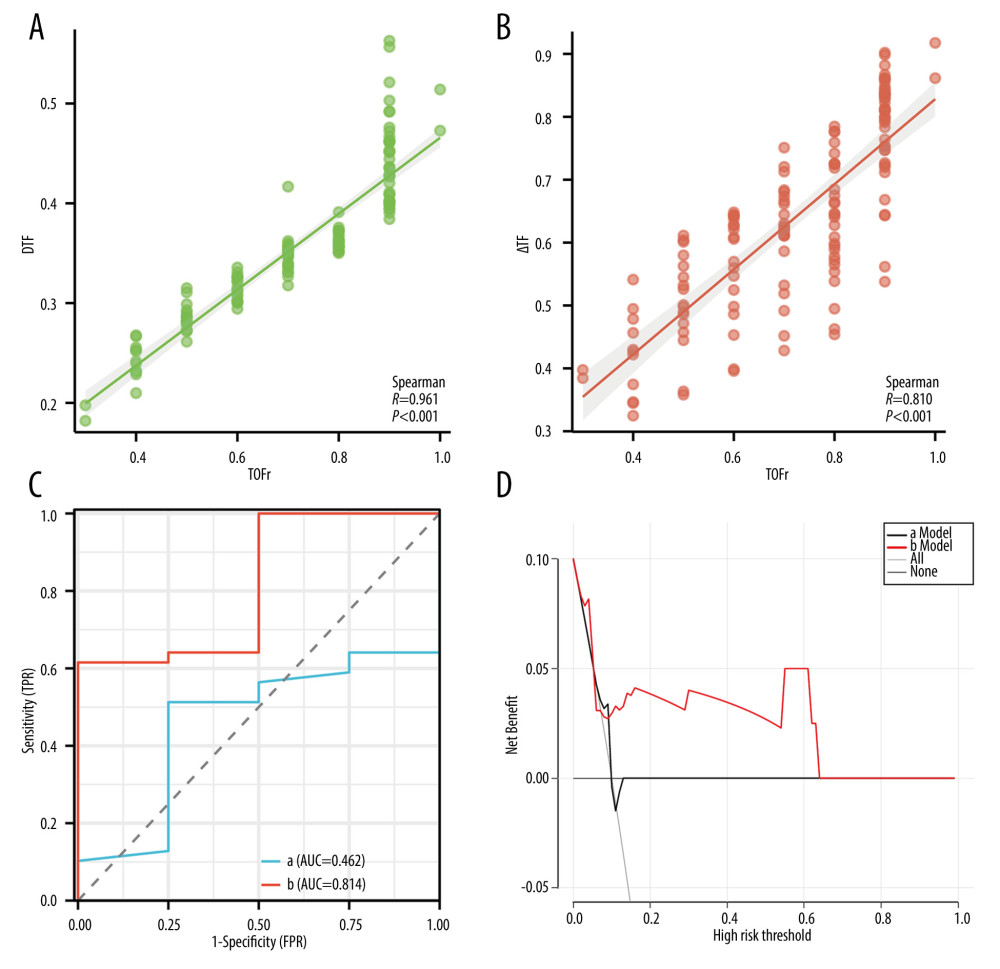
Tables
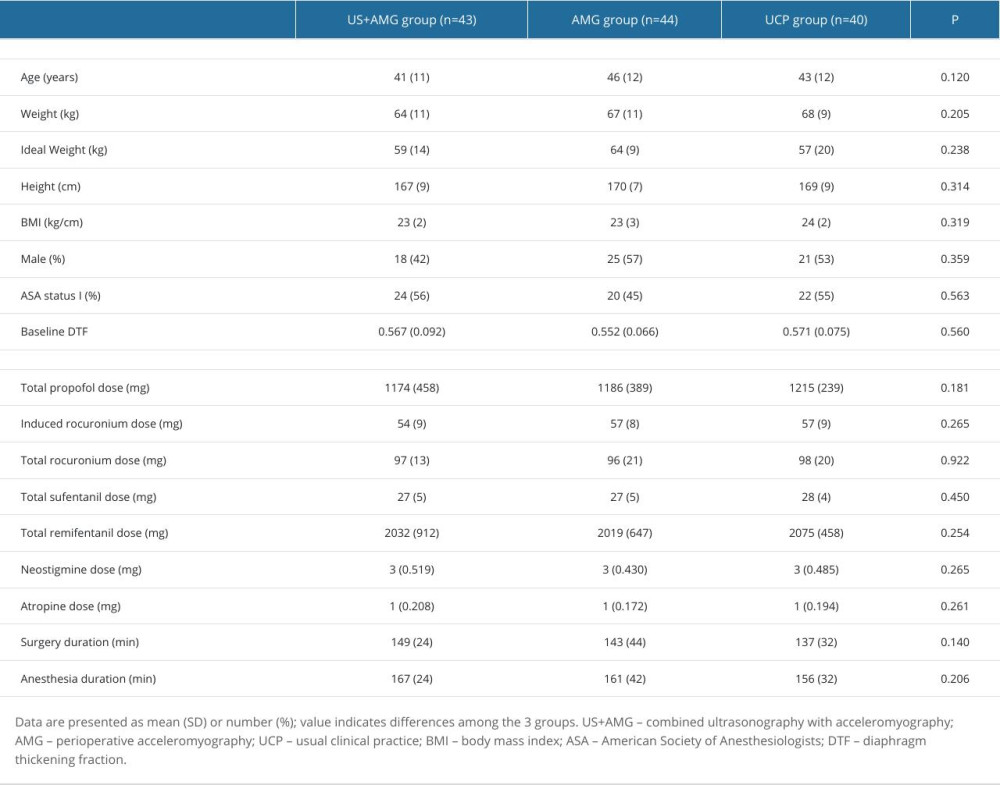
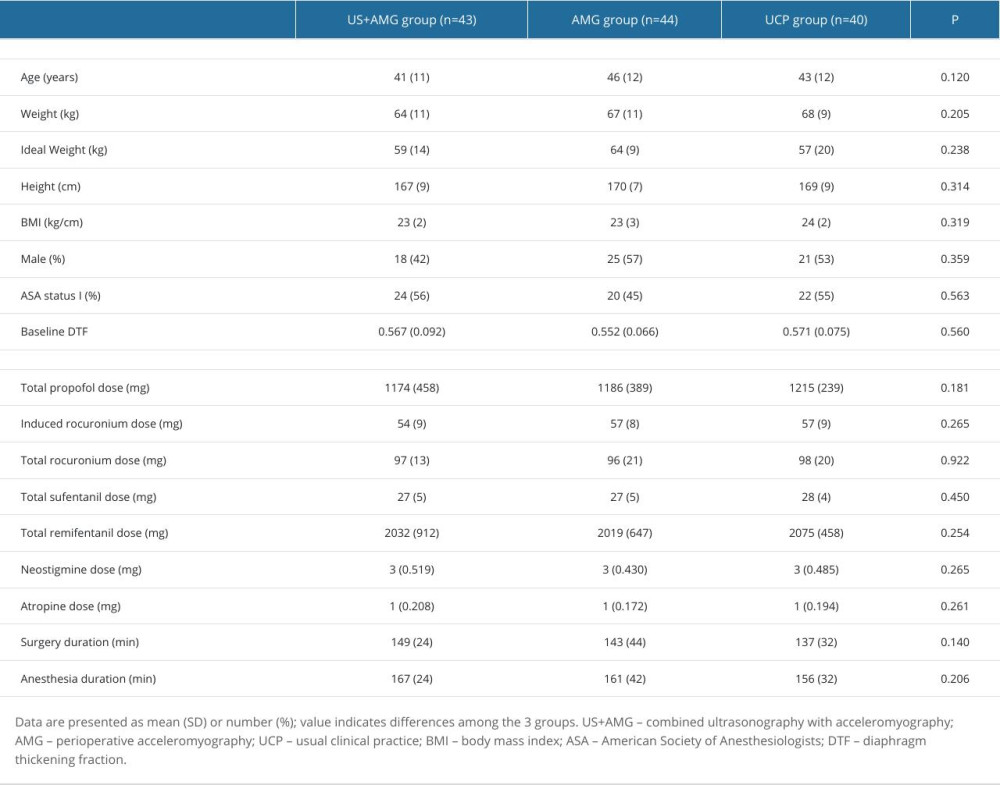
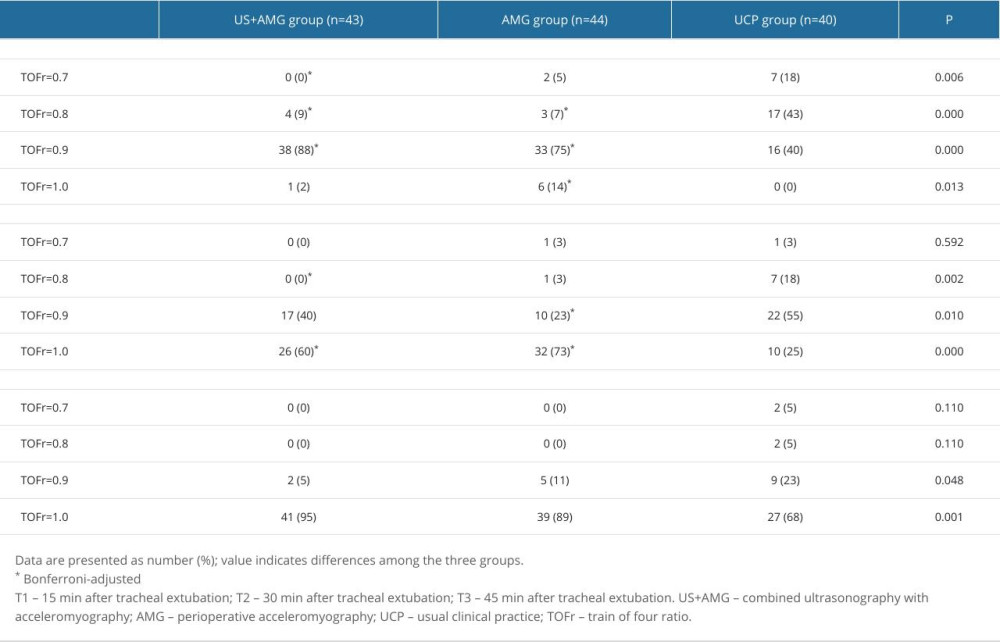
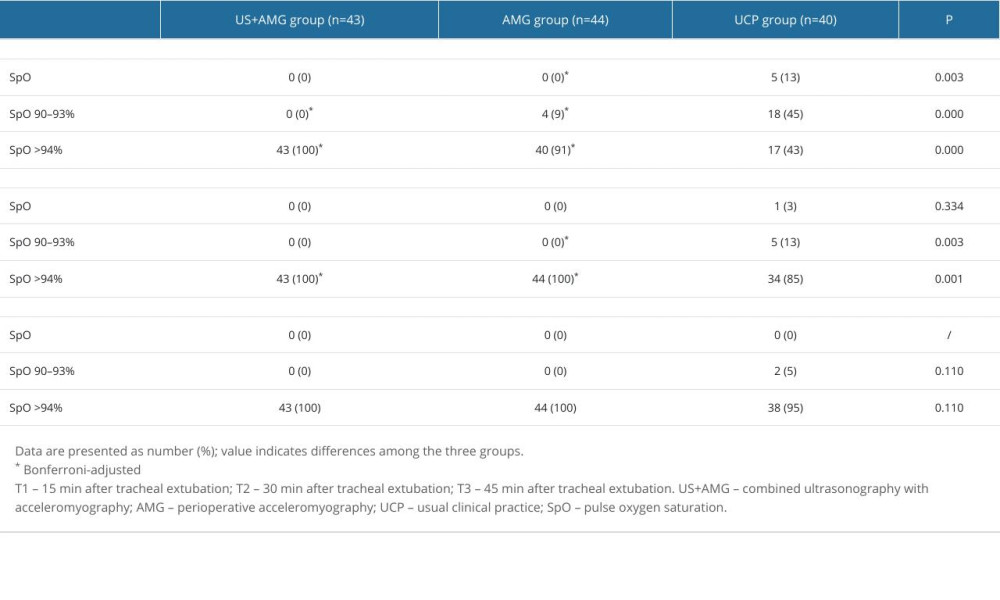
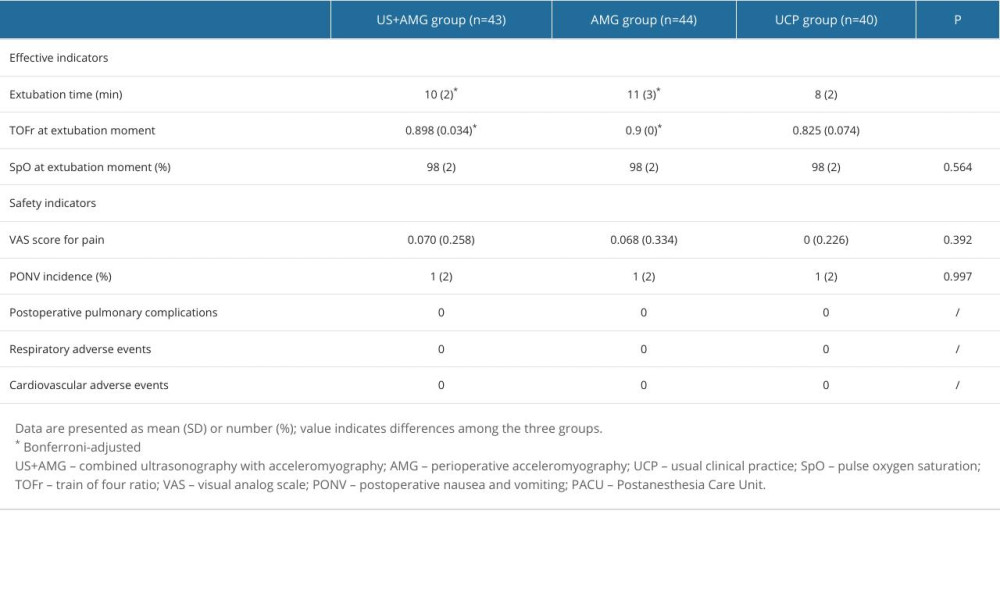
In Press
08 Mar 2024 : Laboratory Research
Evaluation of Retentive Strength of 50 Endodontically-Treated Single-Rooted Mandibular Second Premolars Res...Med Sci Monit In Press; DOI: 10.12659/MSM.944110
11 Mar 2024 : Clinical Research
Comparison of Effects of Sugammadex and Neostigmine on Postoperative Neuromuscular Blockade Recovery in Pat...Med Sci Monit In Press; DOI: 10.12659/MSM.942773
12 Mar 2024 : Clinical Research
Comparing Neuromuscular Blockade Measurement Between Upper Arm (TOF Cuff®) and Eyelid (TOF Scan®) Using Miv...Med Sci Monit In Press; DOI: 10.12659/MSM.943630
11 Mar 2024 : Clinical Research
Enhancement of Frozen-Thawed Human Sperm Quality with Zinc as a Cryoprotective AdditiveMed Sci Monit In Press; DOI: 10.12659/MSM.942946
Most Viewed Current Articles
17 Jan 2024 : Review article
Vaccination Guidelines for Pregnant Women: Addressing COVID-19 and the Omicron VariantDOI :10.12659/MSM.942799
Med Sci Monit 2024; 30:e942799
14 Dec 2022 : Clinical Research
Prevalence and Variability of Allergen-Specific Immunoglobulin E in Patients with Elevated Tryptase LevelsDOI :10.12659/MSM.937990
Med Sci Monit 2022; 28:e937990
16 May 2023 : Clinical Research
Electrophysiological Testing for an Auditory Processing Disorder and Reading Performance in 54 School Stude...DOI :10.12659/MSM.940387
Med Sci Monit 2023; 29:e940387
01 Jan 2022 : Editorial
Editorial: Current Status of Oral Antiviral Drug Treatments for SARS-CoV-2 Infection in Non-Hospitalized Pa...DOI :10.12659/MSM.935952
Med Sci Monit 2022; 28:e935952